As ecological catastrophes go, it’s hard to find anything more bleak or depressing than the coral reef bleaching crisis. We’ve known for 30 years that climate change leads to the death of entire coral reef ecosystems, and yet we’ve done almost nothing to save them.
Fully 16 per cent of the world’s tropical reefs died in 1998, and 2016 was even worse: 70 per cent of the world’s reefs were damaged, some irreparably. Fully 30 per cent of the Great Barrier Reef turned into barren wastelands that year – climbing to 50 per cent of Australia’s crown jewel by the next summer.
So far we’ve lost half the world’s reefs, and one of the biggest threats – climate change – shows no sign of abating. Scientists say if we do nothing, 90 per cent of the world’s tropical reefs will be gone by 2050, along with all the fish, wildlife and humans that depend on them.
“The most shocking thing about watching this crisis unfold was having to hear people say again and again that if we just put big boundaries around the world’s reefs – enclosing them in national parks, marine protected areas and ‘no-take’ areas – they would be fine,” says Dr Ruth Gates, Director of the Hawai’i Institute of Marine Biology.
“Well, 2016 taught us starkly that this isn’t true: that is the best managed reef in the world, and we lost a full third of it in one summer.”
Is there any hope? Yes, says Dr. Gates, if we let go of outdated ideas about wildlife conservation and start to actively intervene. “We have to stop thinking that if we leave nature alone and treat it with the utmost respect that is sufficient. It’s not,” she says.
Not all hope is lost, she says: we just need to apply the science, ingenuity, manpower and – above all – money, while we still can.
“It might seem hopeless – but look at the hole in the ozone layer,” she says. “We identified the problem, acted to remove harmful gases from the atmosphere, and boom: the hole closed. That’s remarkable, and it shows that we can fix these things, we just have to start acting now.”
And the place to start is not with reserves, but with research: understanding the complex biology of these strange animals and finding ways to protect them. “We just need to apply cutting edge science to this,” says Dr Gates.
Why are corals are so vulnerable?
Understanding why corals are so vulnerable to climate change involves understanding a particular quirk of their biology: while most people think corals are plants (or even rocks), they are in fact animals. Not just animals, but clonal organisms that live in clusters of genetically identical units, called polyps. Adding to their complexity, those polyps live in symbiotic relationships with algae that reside in their tissues and photosynthesize sunlight like plants, functioning as tiny cellular batteries. These algae provide up to 90 per cent of a coral’s energy, providing them with both nourishment and pigmentation.
When summer temperatures remain just a few degrees warmer than the normal maximum, stressed corals respond by ejecting their energy-producing, colourful algae. Multi-hued underwater tapestries of branching, bulbous, feathery corals transform within days into barren landscapes of white skeletons. Bleached corals can recover if the water temperature drops within a week or two: the remaining algae can repopulate, and reefs return to good health. But if cooler climes fail to return in time the bleached corals die. Their soft tissues rot away, and white skeletons become smothered with brown seaweed and slime. Fish, turtles and other animals vanish, leaving the grisly graveyard to wither indefinitely. Vibrant ecosystems become dismal wastelands.
The first recorded bleaching took place in Florida in 1911, and again the strange phenomenon was observed in Australia in 1929. It was seen a handful of times for the next half century, until the 1980s when it began to occur with worrisome regularity. By 1990, scientists warned that bleaching was directly linked to climate change, and would only increase in scope and severity, possibly wiping out all reefs within decades. The warnings fell on deaf ears, and catastrophe has struck again and again, just as predicted.
Now half the world’s reefs are gone.
Human assisted evolution
While biologists can’t do much about greenhouse gas emissions, they can help the reefs that remain by applying the exact same strategies we have been using for thousands of years in agriculture and animal husbandry: identify hardy strains, selectively breed them in the lab, and fastidiously cultivate them in the wild.
It makes logical sense: if humans have been able to breed cows, corn, chickens and chocolate for high yields and disease resistance for millennia, why can’t we do the same for coral?
“We simply need to understand what makes some corals thrive, see if that can be applied to other species, and try to develop corals that are bred to be one step ahead of climate change – this is just harnessing basic biology,” says Dr. Gates. “We are simply accelerating what nature can already do.”
One of the most promising areas of research: cultivating strains of coral that are adapted to higher temperatures. At the simplest level this can be done by growing arrays of different species and strains in exceptionally warm tanks of water, identifying the ones that thrive, and then breeding those en masse to out-plant in the wild. “There’s absolutely no reason why this shouldn’t work – and I’ve been surprised at how easy it has been,” says Dr Gates.
In 2015, she published a call-to-arms outline for how to breed such “thermally tolerant” corals in the journal PNAS, subsequently launching a five-year plan to aggressively pursue “human assisted evolution”, funded by Microsoft co-founder Paul Allen.
Understanding how corals function
Breeding the right strains in warm water is just the first step. The next is to go even deeper into understanding the intricacies of coral physiology and working out how to harness this knowledge.
One key area: the microbiome, the enormous range of bacteria, fungi, viruses and other microorganisms that live within the tissues of coral and are integral to their survival. Research into the human microbiome – from the bacteria that live on the surface of our eyeballs to the fungi that dwell within our guts – has exploded in the past decade, and corals are no different than us in requiring an array of symbiotic microbes to survive. Dr Gates has just begun to scratch the surface on the communities of microbes that live within coral, which she described in the journal Trends in Microbiology last year.
Even more tantalising, she says, it might be possible to breed resilience into corals through “epigenetics”: heritable alterations to the ways genes are used, rather than changes to the genes themselves. In 2016, Dr Gates published in the journal Evolutionary Applications preliminary evidence that “DNA methylation” changes can be induced from environmental pressures and then passed onto the next generation.
“If you briefly expose the coral to something that stresses them out a bit but doesn’t kill them, you can build resilience into a species – and that adaptation can actually be passed down through generations,” she says. Early days yet, but cutting edge and promising.
“It’s thrilling to be in a field where you know the work you are doing has a purpose,” says Dr. Gates. “It’s that purpose that keeps me going: we are doing everything we can, and we are trying everything we can.”
Simple techniques can save corals right now
But even without the cutting edge science, there are shockingly simple techniques we can already use to help save coral reefs right now. Dr Gates points to the work of Dr David Vaughan of the Mote Marine Laboratory in Florida who has been working on a technique called “microfragmentation”.
Simply dividing a single piece of coral the size of a golf ball into 25 or even 100 smaller fragments dramatically increases the speed of growth – up to 40 times the normal rate, says Dr Vaughan.
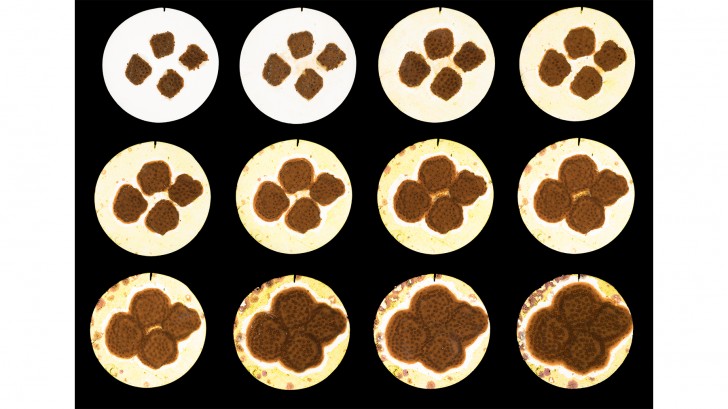
The trick is to arrange those tiny pieces in a grid an inch or two apart – the distance they would grow during that period of accelerated growth. Because coral are clonal animals, microfragments will fuse together when their edges join, forming one single mass of coral.
“Normally it would take a single larvae several years to grow into a piece the size of a golf ball, but if you take a piece of coral the size of a golf ball and cut it into 20 pieces, each the size of a pencil eraser, those each grow into a golf-ball sized chunk in a few months instead of a few years,” he explains. “If they fuse together, you can create a coral head the size of a basketball in just two years – when normally it would take around 75 years.”
Dr Vaughan has not released data on the his coral farms he and his team have planted near Cancun in 2013 using this technique, but their paper is under review and should be published this year. “This restoration project should be one of the first classical success stories that will prove to the world that you can make a difference”
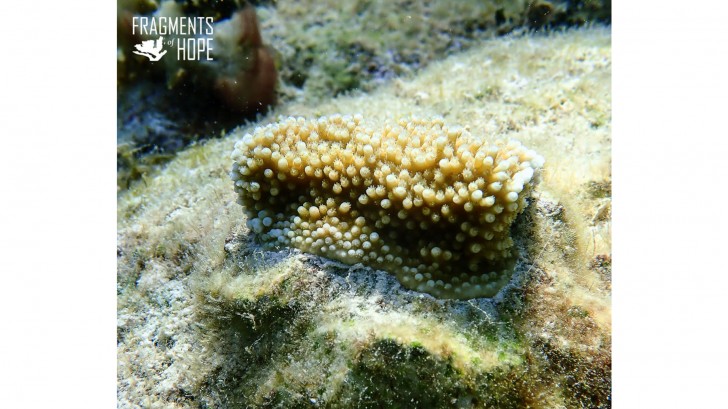
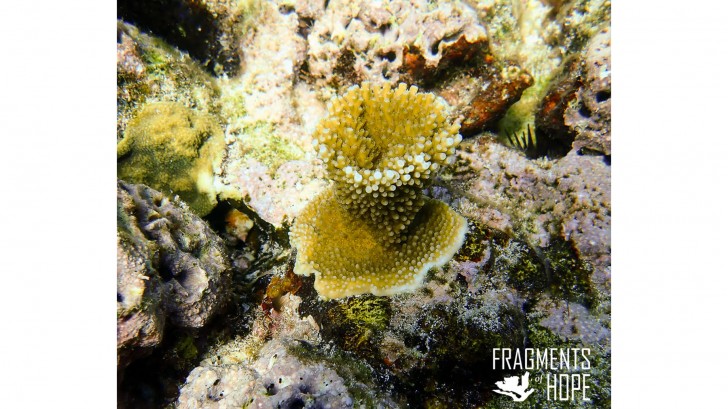
What’s more, some of the elk horn coral (Acropora palmate) they raised in the lab and planted in the wild have grown to sexual maturity and spawned.
Not willing to leave things to chance, Dr Vaughan and his team have cross bred genotypes to produce new genetic combinations. There may be only 25 genotypes in the wild – but in Dr Vaughan’s lab they have created 5,000 new ones which they are testing with high temperature and high acidity (another consequence of high carbon dioxide in the atmosphere) to see which could flourish in our future climate.
Avoiding a coral ‘potato famine’
Having a range of genotypes is crucial if the reefs of the future stand a chance of dealing with other threats: as the saying goes, nature abhors a monoculture.
“If we just propagate the same two or three strains of elk horn, we are just setting ourselves up for the next Irish potato famine – one disease could wipe out everything if they are all genetically identical,” says Dr Jennifer Koss, Deputy Director of the National Oceanic and Atmospheric Administration’s Coral Reef Conservation Program. “We need to make sure we have a diversity of species, and diversity within species, so future reefs will have tolerance not just to heat, but emerging pathogens.”
For decades, scientists have predicted that climate change would lead to the rise of new epidemics as infectious agents are spread into new environments, and already a new coral disease is spreading through the Florida keys: a strange white plague. Biologists know almost nothing about it so far – they don’t even know if it is a virus, fungus, bacterium, or something else.
“We are watching it and trying out different solutions, such as looking at embedding antibiotics into a paste – but at this moment we don’t even know if it’s one single disease or several moving together,” says Dr Koss. “All the global stressors are only getting worse, but on the bright side our growing techniques are pretty solid, including Dr Vaughan’s microfragmentation technique, which is a major step forward. Now we need to figure out how to scale up that technique and get the corals out onto reefs in configurations where they will reproduce on their own.”
Physical restoration
Which is why Frank Mars has been focusing his efforts on what he calls “the physical side” of coral restoration: the right configurations that allow them to take off on their own.
“Most people are just so preoccupied with farming living coral and gluing them out onto reefs, they haven’t given any thought to what they are sticking them to,” he says. New coral plantations cannot be built where there was no reef before, such as on sand, he says. Nor can resilient coral be grown on floating nurseries at the ocean’s surface, as is being done around the world.
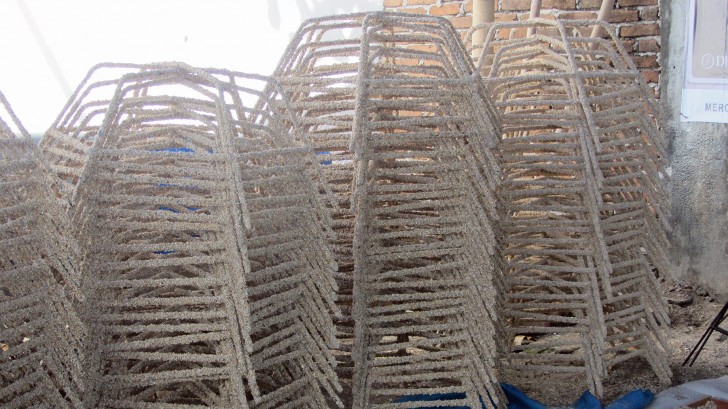
Mars’s solution is deceptively simple: hexagonal steel structures, propped up on legs, dubbed “coral spiders”.
So far Mars’s Coral Reef Rehabilitation Project has installed over 8,600 spiders covering more than 8,000 square metres of ocean floor in Indonesia. By laying hundreds of these and linking them together on the sea floor, he says he can create not only the appropriate surface small corals can be attached to, but also create the appropriate three dimensional structures that will attract fish – crucial for maintaining the health of an entire reef.
“The key to managing a reef is to manage the green algae that will naturally grow on the surface of the coral – and the key to that is maintaining populations of herbivorous fish that will eat the algae,” he says. “If you look at most coral gardens around the world, caretakers have to spend all their time scrubbing coral with toothbrushes because they haven’t built the right habitat for fish.”
The difference with his steel spiders, says Mars, is that they create protective shells where smaller fish can hide from larger fish, enticing them to stay and kickstarting the regeneration of the entire ecosystem and its vast assemblages of species.
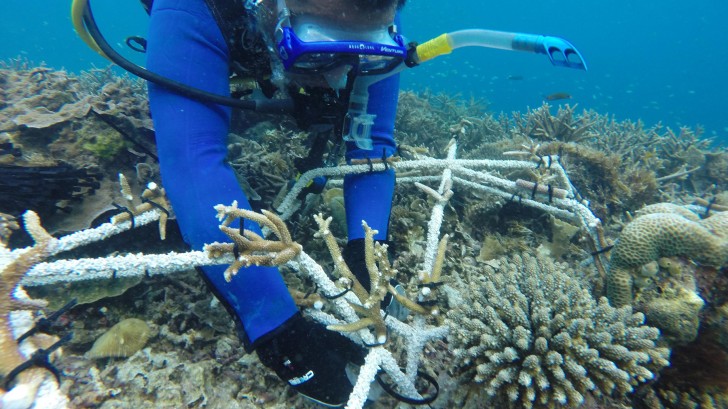
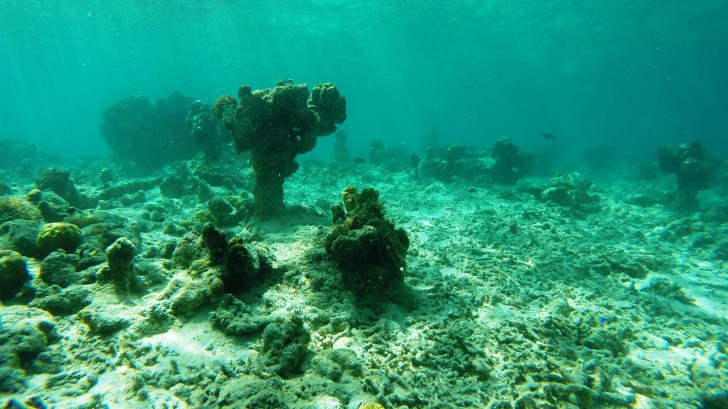
“You can actually see and hear the reef come to life year on year,” he says. “We actually see turtles, sharks, and tuna return – communities in the tropics can fish again on reefs that had been destroyed.”
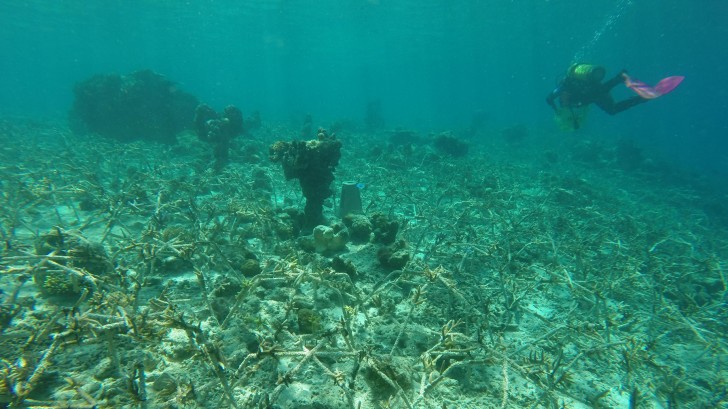
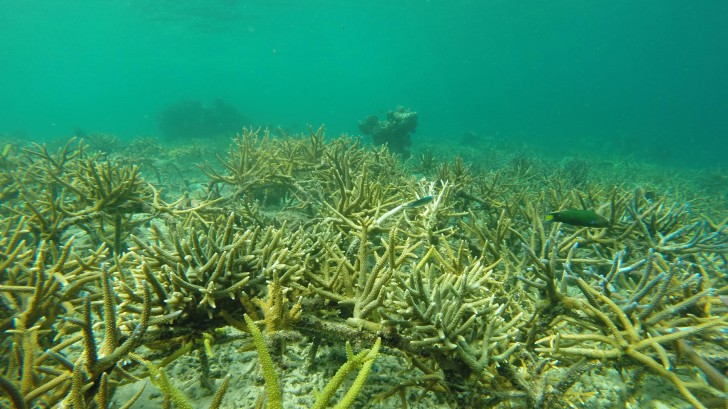
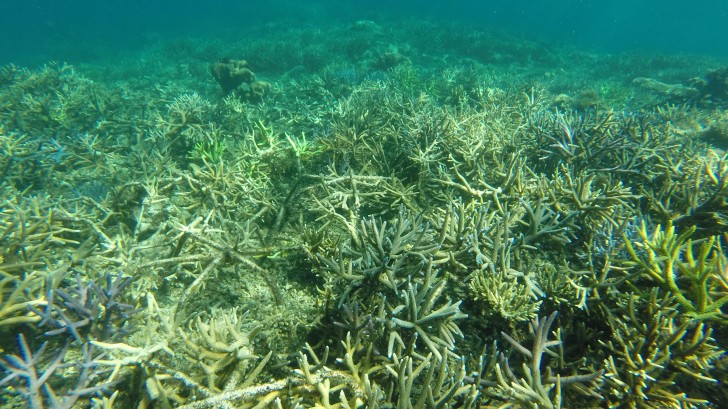
Some think we should go even further than simply installing naked steel cages.
By flowing low voltage direct current electricity into rebar structures, proponents of the Biorock technology say they can increase the rate that minerals are taken from sea water by growing coral, which they use to build their limestone skeletons.
Biorock structures were initially constructed in the 1970s by architect Wolf Hilbertz as a means to build complex, growing structures in the ocean; biologist Dr Tom Moreau adopted the technology to facilitate the growth of corals and other organisms that build shells and skeletons using minerals from sea water, such as oysters.
“With our method, the electrical field stimulates biochemical metabolism: the production of ATP, the fundamental energy of life,” says Goreau. “This makes a spectacular difference.”
Rates of growth vary from species to location, but a 2014 study published in the journal Natural Resources, reviewing all the Biorock structures built since 1976, asserts that on average corals grow about 3-4 times faster with a gentle electric current. In times of catastrophe, such as after bleaching or a hurricane, Dr Moreau say corals grown on Biorock have survival rates far higher. Following the 1998 bleaching crisis in the Maldives for example, he says corals on Biorock structures had survival rates 16 to 50 times higher than on surrounding reefs.
Even more impressive, says Goreau, is the rate of “settlement” on Biorock: the appearance of brand new coral colonies from free-floating larvae that attach onto the metal structures without human intervention. Larvae settle onto electrically charged Biorock at a rate almost 26 times higher than uncharged metal.
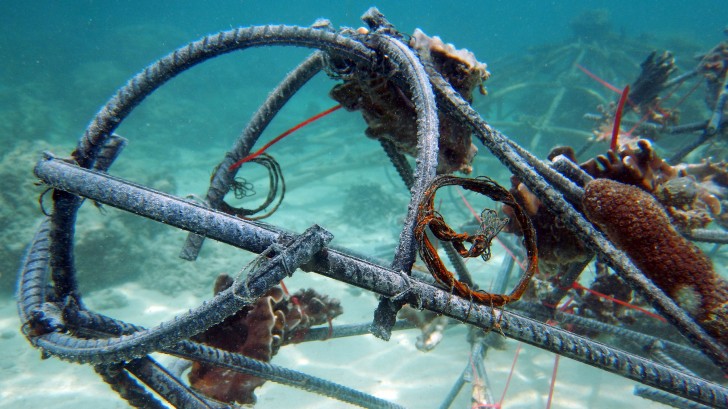
Electricity on Biorock constructions – now numbering close to 500 worldwide, 400 in Indonesia alone – is typically delivered by mains electricity from the grid, but solar panels obviously provide a better solution. “The dream is to supply power with marine turbines,” says Delphine Robbe, who has worked for Biorock for 15 years in Indonesia, and fully believes in the technology. “It creates a bubble of survival in times of stress.”
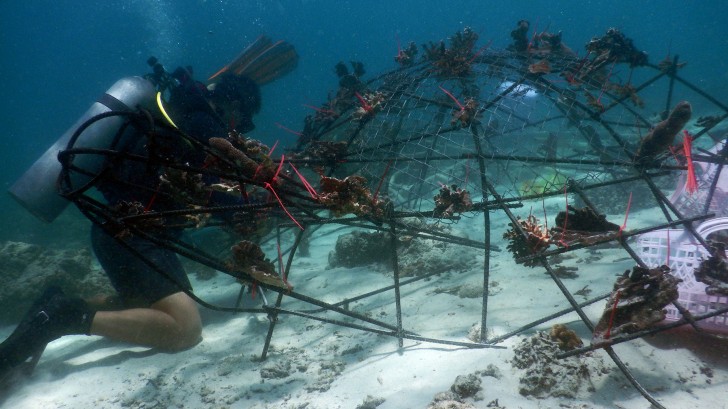
However not everyone is convinced that low voltage electricity, cables, solar panels, wave turbines, infrastructure and maintenance, are necessary to regenerate a reef. Some have suggested Biorock technology works for the same reason Frank Mars’s spiders work: by creating three dimensional structures that crate habitats for fish and foster the growth of the whole ecosystem.
However everyone is in agreement with Goreau about two things:
One: “We reached the tipping point for coral bleaching in the 1980s – now we have lost 30 years when something should have been done,” he says.
And, two: “There’s just no money for this – we have had to operate for 30 years without funding.”
Funding the reef
The lack of money devoted to research, restore and preserve coral reefs is possibly the most perverse aspect to the entire tragedy. Unlike cutting down the Amazon rainforest or blowing the tops off mountains to mine for coal, humans did not intend to destroy the world’s coral reefs. But we have known for 30 years that they are vanishing before our eyes, and have done close to nothing to save them – not even taking appropriate measures to improve water quality. Even if water temperatures fall after a bleaching event, corals in polluted areas struggle to recover – such as the Caribbean coral ecosystem, which has been almost entirely annihilated.
“The ultimate challenge is who is going to fund the rebuilding of the reefs?” asks Frank Mars – a member of the Mars family (as in the chocolate), the third richest family in America. “I have had the budget and the freedom to experiment with different approaches and work on this problem for 15 years. But philanthropy alone cannot solve this problem.”
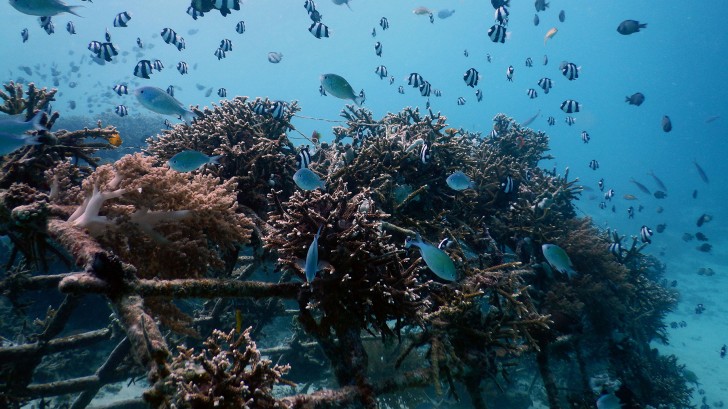
Dr Gates in Hawaii agrees. “For years I had to stagger on without funds – federal agencies were never keen to fund coral research, either because they didn’t understand the importance of it, or because they were never big on funding cutting edge science where the outcome is unknown. Fortunately, philanthropists have stepped in,” says Dr Gates, whose work is funded by Paul Allen, co-founder of Microsoft. “But we urgently need more money – no two reefs in the world are the same, so we have to try a lot of things in a lot of different places – and that’s just not possible without the funds.”
The lack of any international effort to fund coral preservation does not make for cheerful reading, but a ray of hope can be found in the price tag of coral preservation: all signs indicate it would be astonishingly cheap.
“People have talked about ‘billions’ of dollars, but I think that’s a fancy number. Literally for $1m or $2m I could take elk horn coral off the endangered species list in Florida within just a few years,” says Dave Vaughan, who believes his simple microfragmentation technique is ready to change the world.
“How many organisms can you say that for? Not many. If somebody asked me if I could take the polar bear off the endangered species list for $100m, I would have to say no, I can’t do it. But a species of coral? I’d say $10m, tops – and I can do it in two years.”
The question clearly is not if we can save the coral reefs, but if we choose to.